This year’s Nobel Prize curtain has drawn to a close, with the pioneers of mRNA vaccine technology clinching the 2023 Nobel Prize in Physiology or Medicine. However, for many, there remains one technology that is seen as a ‘missed gem’ of the Nobel Prize.
Light, as a form of electromagnetic radiation, profoundly influences various aspects of life on Earth, such as photosynthesis and circadian rhythms in biological processes. This visually dazzling and colorful yet seemingly intangible light is both mysterious and captivating. In the development of neuroscience, light plays a significant role, which can be broadly categorized into three aspects. Firstly, the application of Green Fluorescent Protein (GFP), which earned the 2008 Nobel Prize in Chemistry, allows researchers to label neurons with fluorescence. Secondly, by coupling voltage-sensitive proteins on cell membranes or calcium-sensitive proteins inside cells with fluorescent proteins, researchers can monitor neuronal activity through changes in fluorescence. The third aspect is the use of light to manipulate neuronal activity, known as optogenetics.
Back in 1979, Francis Crick, who, along with James Watson, unraveled the double helix structure of DNA and was a co-recipient of the 1962 Nobel Prize in Physiology or Medicine, posited a major challenge facing neuroscience: the ability to control a specific type of cell in the brain without affecting others. Before the advent of optogenetics, physical or chemical means were primarily used to control cells. Unfortunately, existing electrodes could not precisely target specific cell groups, and the action of chemical drugs was too slow. It was not until the early 21st century that the spark of optogenetics illuminated the entire field of neuroscience. Researchers now simply need to shine light to control a tiny molecular switch, allowing them to swiftly activate or deactivate specific neurons with a ‘click’.
1. Optogenetics Hall of Fame
The Photoreceptive Elements Discovered in Bacteria
The story begins over half a century ago. In 1969, 29-year-old Dieter Oesterhelt, fresh from his doctoral graduation, began his postdoctoral research at the University of California, San Francisco. His project focused on a type of bacteria that thrived in high-salinity conditions, known as Halobacterium salinarum, specifically studying its purple membrane structure. During this pursuit, Oesterhelt encountered what would become his lifelong academic muse: bacteriorhodopsin. He discovered that this protein, located in the cell membranes of Halobacterium salinarum, functioned as a light-sensitive ion pump. Rapidly activated by light, this protein drove proton transport, generating chemical energy and thus enabling the bacteria to survive in low-oxygen conditions by harnessing light.
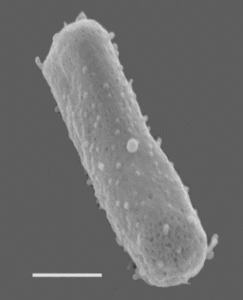
Caption: Halobacterium salinarum NRC-1 (Scale = 270 nm). Figure Source: Wikipedia
Subsequently, Peter Hegemann, a student of Dieter Oesterhelt, continued the legacy of his mentor. With Dieter Oesterhelt, he isolated another type of light-sensitive ion pump, specifically the chloride ion-specific halorhodopsin, and also extended the exploration of photoreceptors to other biological systems, including Chlamydomonas reinhardtii. Building on his extensive research experience, Hegemann astutely hypothesized that the phototactic behavior of Chlamydomonas reinhardtii might be linked to a type of light-sensitive protein.
After decades of diligent research, Peter Hegemann and his team, at the beginning of the 21st century, successfully identified Channelrhodopsin-1 (ChR1) and Channelrhodopsin-2 (ChR2). Upon exposure to light, these proteins induce cell depolarization, generating a photocurrent that ultimately triggers the phototactic movement of the flagella in Chlamydomonas.
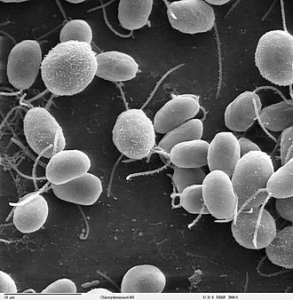
Caption: Chlamydomonas reinhardtii. Figure Source: Wikipedia
The photoproteins described earlier, namely bacteriorhodopsin, halorhodopsin, and channelrhodopsins, have found applications in optogenetics. Naturally occurring bacteriorhodopsin, the first discovered member of this family that pumps protons out of the cell, and halorhodopsin, which pumps chloride ions into the cell, typically have inhibitory effects in the nervous system. This is due to the hyperpolarizing currents generated by these two proteins, which make it harder for neurons to fire action potentials. Conversely, naturally occurring channelrhodopsins, which predominantly allow positively charged ions to flow through the protein pores, tend to depolarize and excite neurons.
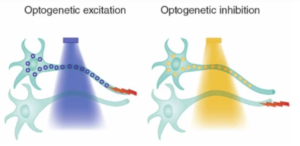
Caption: Targeted activation (like activating channelrhodopsin with blue light) or inhibition (such as activating halorhodopsin with yellow light) enables rapid, cell-specific, and even projection-specific neuronal manipulation. Figure Source: Reference 1
Passing the Torch Among Three Forces of Power
The discovery of photoproteins may have laid an excellent foundation for the birth of optogenetics. However, the integration of these photoproteins with the manipulation of neurons still required a final, pivotal push from the trailblazers of the era. As time marched into the late 20th and early 21st centuries, three key forces played an especially vital role in propelling the emergence of optogenetics.
The first of these forces originated from the team of Austrian neuroscientist Gero Miesenböck. Miesenböck envisioned using photoproteins to manipulate neurons, but initially, he struggled to find an efficient molecular tool among them. It was not until 2002 that Miesenböck’s pioneering work demonstrated the heterologous expression of three photoreceptor genes from fruit flies in vertebrate neurons, enabling activation of specific neuron types through light stimulation.
Another pivotal group was led by Peter Hegemann and his collaborator Georg Nagel. Between 2002 and 2003, Nagel and Hegemann identified the key channelrhodopsins, ChR1 and ChR2, involved in the phototactic behavior of Chlamydomonas reinhardtii. They innovatively expressed these proteins ectopically in cells of other species, proving that their ion channel activity remained light-activated even when expressed heterologously. This method required only the expression of a single protein. The work of Nagel and Hegemann provided an incredibly simple and effective molecular tool for optogenetic techniques.
The final pivotal contribution came from the team of Edward S. Boyden and Karl Deisseroth, who masterfully integrated ChR with neuronal manipulation. In 2005, Boyden and Deisseroth reported a landmark study: by expressing just a single molecule of ChR2 in hippocampal neurons, they could trigger action potentials in specific neurons with millisecond precision through light, elegantly establishing the fundamental paradigm of optogenetics. Moreover, Boyden and Deisseroth’s team refined this tool, enabling researchers to rapidly and precisely silence specific neurons.
In 2006, Karl Deisseroth officially coined the term ‘optogenetics’ for this tool. Optogenetics was rapidly adopted across various fields of biology due to its groundbreaking potential.
Major Awards in Optogenetics in Recent Years
In 2018, Peter Hegemann, Edward S. Boyden, and Karl Deisseroth were awarded the Canada Gairdner International Award.
In 2019, Peter Hegemann, Gero Miesenböck, Edward S. Boyden, and Karl Deisseroth received the Warren Alpert Foundation Prize.
In 2020, Peter Hegemann, Georg Nagel, and Gero Miesenböck were honored with the Shaw Prize in Life Science and Medicine.
In 2021, Dieter Oesterhelt, Peter Hegemann, and Karl Deisseroth were recipients of the Lasker Awards for Basic Medical Research.
Regrettably, Dieter Oesterhelt passed away in 2022.
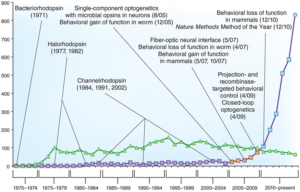
Caption: As of 2015, a timeline of publications on photoproteins and optogenetics research. In recent years, the number of studies related to optogenetics has surged dramatically. Figure Source: Reference 12
2. Optogenetic Methodology Explained
Optogenetics allows for the manipulation of complex biological systems, such as freely moving mammals, with millisecond precision and cell type-specific accuracy using light. This remarkable technique involves three core components: (a) the family of photoproteins, which undergo structural changes upon light stimulation, triggering ion flow across cell membranes; (b) targeting the expression of specific rhodopsin proteins in certain cell types within specific brain regions; and (c) applying light stimulation with spatial and temporal precision to the targeted brain areas and cell types.
In a typical optogenetic experiment, the following six steps are primarily involved:
Firstly, researchers construct an expression vector containing both the ChR2 gene (or other photoproteins) and genetic elements to control its expression, such as cell type-specific promoter sequences.
Next, the expression vector is packaged into a virus.
Subsequently, this virus is injected into the animal’s brain. Although the virus infects neurons broadly, the rhodopsin proteins are expressed only in subpopulations of cells with the necessary machinery to activate the specific promoters. This results in cell type-specific expression of the light-sensitive rhodopsin proteins, which localize to the cell membranes of these neurons.
Fourthly, an optic fiber is implanted into the animal’s skull.
Fifth, light of a specific wavelength is transmitted through the optic fiber to activate these rhodopsin proteins.
Finally, researchers record and analyze the resulting electrophysiological and behavioral data.
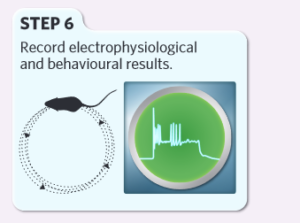
Caption: Basic experimental steps of optogenetics. Figure Source: Reference 15
Caption: Basic experimental steps of optogenetics. Figure Source: Reference 15
3. How Does Optogenetics Work?
In 2010, optogenetics was hailed as the ‘Method of the Year’ by Nature Methods and was recognized by Science as one of the breakthroughs of the decade. The application of this technology spans two main areas: basic research on the function of neural circuits and clinical treatment of specific diseases.
Basic Research – Discovery of the ‘Success Breeds Success’ Circuit
The first paper, published in 2007, on the application of optogenetics in animals, studied the function of neurons secreting hypocretin in the lateral hypothalamus. Researchers stimulated the activity of hypocretin-secreting neurons in the lateral hypothalamic area using optogenetics, clarifying their role in the sleep-wake state transitions in mice.
Optogenetics has also been applied to elucidate the physiological mechanisms underlying various psychiatric disorders. For instance, it has elegantly demonstrated the crucial role of cholinergic interneurons in the nucleus accumbens in cocaine-conditioned responses, the importance of midbrain dopamine neurons in chronic mild stress-induced depressive-like phenotypes, and the role of cortico-striatal neural circuits in mechanisms of compulsive behavior, as well as the involvement of the neural circuit from the medial prefrontal cortex to the basolateral amygdala in anxiety.
In 2017, Hailan Hu’s team at Zhejiang University notably used optogenetics and other techniques to validate the ‘winner effect’ in mice. They showed that the neural circuit from the dorsomedial hypothalamus nucleus to the prefrontal cortex mediated long-term changes in social dominance status in competitive scenarios, influenced by past experiences of victory.

Caption: Optogenetics Empowers the ‘Underdog’ – Light stimulation of the thalamo-prefrontal cortex neural circuit boosts the fighting ability of mice previously disadvantaged in competitive situations. Figure Source: Reference 18
Clinical Treatment – ‘Restoring Sight’ with Phototherapy
In 2021, a study published in Nature Medicine showcased, for the first time, the application of optogenetics in treating a neurodegenerative eye disease, retinitis pigmentosa, achieving partial restoration of visual function in patients. In this experiment, researchers injected a virus carrying photoproteins into the eyes of patients blinded by this disease. Subsequently, the patients’ eyes were exposed to light stimulation, activating the optogenetically modified retinal ganglion cells.
Following this treatment, patients regained the ability to perceive, locate, count, and touch various objects using their vision. Multi-channel electroencephalogram recordings during this visual perception process revealed object-related activity in the visual cortex, indicating a partial recovery of visual function in these patients.
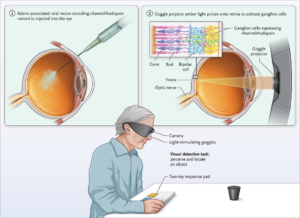
Caption: Optogenetics ‘Restores Sight’ in the Blind. Figure Source: Reference 23
As highlighted in Nature Methods, using light to regulate specific activities in specific cells has achieved tremendous success in biological research, illuminating previously unexplored paths in science.